Heavy Industry
Heavy industry is responsible for 1/3 of global GHG emissions.
Given that the average economic lifetime of industrial facilities is 20 years or more,
this indicates all new investment must be net zero emitting by 2035–2060
or be compensated by permanent, verifiable and transparently accounted negative emissions to guarantee GHG-neutrality.
Before the Paris Agreement was adopted in 2015, heavy industry was often characterized as a “hard to abate” sector. Industrial GHG mitigation studies were mainly focused on energy efficiency, coal-to-gas or electricity fuel switching, and carbon capture and storage (CCS). In projection exercises simulated by global models, industry was typically allowed to maintain roughly half its emissions out to 2050, compensated by negative emissions from bioenergy combined with CCS in the power sector. The ratification of the Paris Agreement and the adoption of global net zero targets utterly changed the industrial mitigation discussion, sending analysts, modelers, engineers, and company strategists back to the drawing board. Reducing industrial GHG emissions to Paris Agreement compatible levels is now regarded as both technically possible and politically feasible, but requires major process changes. Most of the technology needed already exists at the pilot to near commercial level but requires both a fundamental change in the approach to industrial demand and production and a systemic uplift in policy stringency.
2020
Dr Chris Bataille
June 2nd 2022
Dr Chris Bataille and Seton Steibert P.Eng
December 6th, 2022
Seton Stiebert P.Eng and Dr Chris Bataille
Methodology
A systemic, multi-strategy approach to decarbonizing industrial electricity and heat use as well as eliminating process emissions, based on the industrial mitigation section of the IPCC’s “Climate Change 2022: Mitigation of Climate Change” report is illustrated below:
Source: Bataille, Chris, 2022, “Industry Emissions: Process Changes and Policy Options on the Road to Net Zero”, Commentary for Columbia SIPA Center on Global Energy Policy https://www.energypolicy.columbia.edu/publications/industry-emissions-process-changes-and-policy-options-road-net-zero/
Demand and material efficiency: Material efficiency can be achieved through improved designs and building codes, incorporating greenhouse gas (GHG) considerations. This could cut down cement and steel usage by 26% and 40% respectively.
Material Circularity: Enhancing material circularity via high-quality recycling is crucial for reducing emissions from metals, plastics, and eventually, chemicals and concrete aggregates.
Energy Efficiency: Energy efficiency should maximize end-use efficiency (such as using suppling low temperature heat from industrial heat pumps) and also systemic efficiency (such as streamlining processes or process integration to reduce overall energy requirements).
Electrification and Fuel Switching: switching to clean electrification for low temperature heat is key, with synthetic renewable fuels for high temperature processes. Synthetic net-zero GHG fuels and feedstocks made with low-GHG elements can already replace fossil fuels, but are currently more expensive.
Process Changes: Implementing process changes to utilize zero-emissions fuels and feedstocks is vital. Innovations in steel and chemical production are emerging, which use clean electricity, hydrogen, and low-GHG carbon.
Carbon Management: Carbon management, including capturing and utilizing CO2, sourcing low-GHG carbon sources like biomass, and geological storage is likely necessary, especially in the cement industry.
Policy makers seeking pathways toward industrial decarbonization could consider the following options:
- Enact policies to ensure a large, growing, reliable, and relatively inexpensive supply of very low GHG electricity.
- Speed up the process of innovation and early-to-late commercialization for near-zero-emissions technologies to reduce production investment risk, with a focus on establishing lead markets
- Establish physical industrial clusters to reduce the cost of blue and green hydrogen and CCS, and to allow waste heat reuse with industrial heat pumps.
- Implement policies to drive broad market uptake of near-zero industrial options, including: loans and tax credits to alleviate CAPEX-heavy investments, such as heat pumps; GHG standards for heat and steam; carbon pricing; and performance regulations.
- Establish international coordination regarding green procurement, GHG accounting, trade policies and technology transfer and finance for developing countries, where most new demand and investment will be in the coming decades
Key Findings
Industrial decarbonization technology review.
General results
Options for industrial decarbonization can be organized into a decision tree. The first step is to reduce end-use demand through sustainable consumption patterns and substitution of GHG intense materials. The next decision is whether to keep the existing process or commercialize alternative processes. If the existing process is kept, there is a choice between carbon capture and utilization/storage or using a GHG free heat source. If new processes are chosen, options include replacing fossil fuels and feedstocks with biomass, electrification, hydrogen, and synthetic hydrocarbons. Industrial symbiosis is another option. Carbon capture is viable but expensive for industrial use. Work is underway to reduce costs through advanced membrane separation technology and chemical looping.
The pulp and paper industry: key to a biomass pathway?
The need for pipelines to transport CO2 for storage put infrastructure limitations on the use of all types of Carbon Capture and Storage (CCS) globally. For many existing facilities, their supply chains and markets are simply not where the geological disposal is, and transport and more critical rights of way will be needed.
Keystone chemicals & renewable fuels: decarbonized electricity and biomass
Bio-liquids, bio-gases, and bio-solids are considered as a viable alternative to fossil fuels because of their carbon neutrality. However, biomass faces significant challenges such as competition for land use, biodiversity conservation, life-cycle emissions, security of supply, and air quality. Hydrogen can replace fossil methane for process heat and feedstock and can be produced through various methods. Renewable hydrogen, gasified or digested biomass, and CO2 capture can produce synthetic renewable hydrocarbons and other vital chemicals. Methane, methanol, and ethanol can be made from biomass through anaerobic digestion or gasification, but these technologies are not currently cost-competitive without market interventions or carbon pricing.
Iron & steel, extractive metallurgy & glass: decarbonized electricity or CCUS
The iron and steel sector emits significant GHG emissions, with the blast furnace-basic oxygen furnace (BF-BOF) route being the biggest culprit. Decarbonization can be achieved through the use of renewable hydrogen in a direct reduced iron-electric arc furnace (DRI-EAF), electrowinning, or electrolysis of iron ore. EAFs can also melt recycled steel for reuse, but impurities and limited economic viability are obstacles that need to be addressed. Recycling systems and products designed for easy disassembly are needed to improve the process.
Glass
Glass production is process heat intense and therefore GHG intense today. The two pathways to decarbonization cited by the literature, discussed earlier in the general results, were oxy-combustion with CCUS and direct electric melting (Ricardo-AEA Ltd., 2013; WSP Parsons Brinkerhoff and DNV GL, 2015).
Cement: increasing cementious materials, CCUS and future chemistries
Cement production is a major source of GHG emissions and reducing emissions requires using less clinker and more cementious materials. Material substitution can also reduce emissions by 20-60%. Carbon capture, utilization and storage (CCUS) is a way to lower emissions during the cement production process, and alternative chemistries for cement are being piloted that could potentially reach net zero or negative emissions. However, these alternative chemistries would require substantial testing and market education before being fully commercialized.
Policy package design
Policy package design for transitioning to zero carbon industry
The article discusses the challenges of implementing emerging technologies to reduce greenhouse gas emissions in the industrial sector. The high production costs and lack of market incentives make it difficult for firms to transition to low-carbon technologies. The authors suggest a policy framework that includes commitments, technology uptake encouragement, niche development, and supporting institutions to facilitate a transition to zero or negative carbon technologies.
Penetration of near-commercial net zero technologies
Carbon pricing is seen as a key policy tool to encourage low greenhouse gas (GHG) innovation and discourage carbon-intensive activities. The materials sector, which includes steel and cement production, requires a strong role for carbon pricing to facilitate a transition to CO2-efficient material production, substitution, recycling, and end-use efficiency. Various methods exist to internalize carbon costs in decision making, such as direct carbon pricing, performance-based regulations, and end-use consumption carbon pricing. However, the complexity and heterogeneity of industrial production processes, value chains, and end-uses argue for a combination of production and consumption pricing. Border-carbon adjustments (BCAs) are one way to equalize emissions pricing for imports and domestic production, but they face practical difficulties and must be compliant with WTO rules.
R&D, piloting & demand support for emerging technologies & processes
Numerous emerging technologies need significant support to evolve to commercial availability in time to help meet the Paris Agreement goals. These include lower-cost and more efficient electrolysis, post-combustion and direct air CO2 capture, biomass gasification, and solid oxide fuel cells. Firms attempting to commercialize these technologies will likely incur substantially higher production costs with few monetizable co-benefits. Governments can use their purchasing power to create a market pull for low carbon products and commodities using carbon content-based procurement regulations. Industrial R&D, piloting of net zero technologies, and demand support would also ideally be pooled across technology/project portfolios and amongst firms and government entities to allow global collective learning and risk and cost diversification.
Supporting institutions
Several institutional actors need to be created to support the market and coordinate the R&D, commercialization, and market uptake processes for the transition to a low-carbon economy. These institutions include those for coordinating research and technology diffusion, planning for industrial parks, training the labor force, investing in necessary infrastructure, and developing a regulatory and monitoring framework. The measurement of GHG intensity of intermediate and final products is also crucial for implementing financial carbon pricing and declining output subsidies based on GHG intensity.
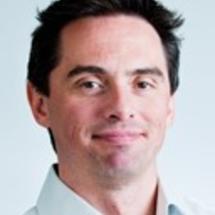
Dr. Chris Bataille
Associate Researcher, IDDRI.org, Paris / Adjunct Professor, Simon Fraser University, Vancouver, Canada
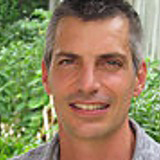
Seton Stiebert
Professional Engineer, Associate International Institute of Sustainable Development (IISD), Ottawa, Canada
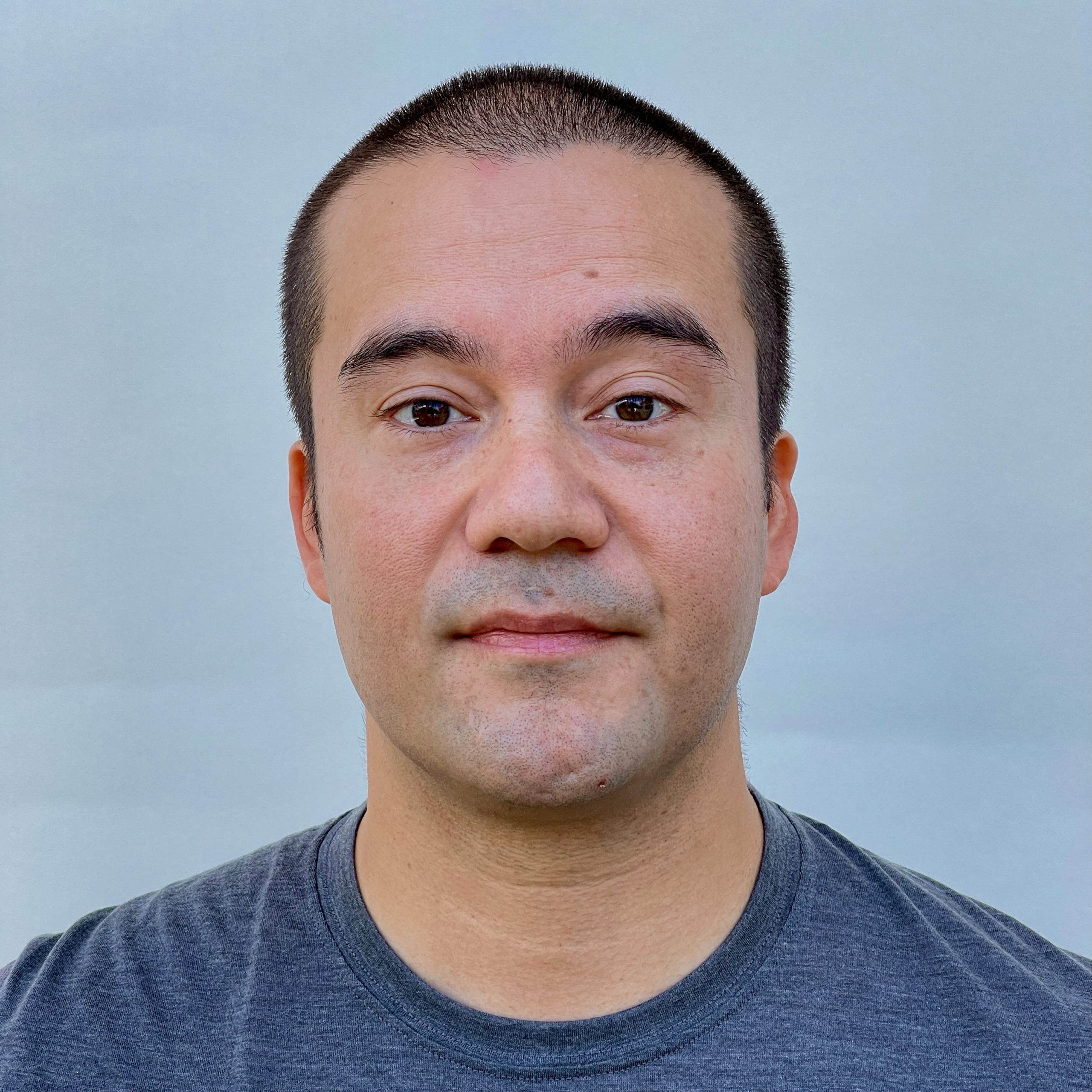
Dr. Francis Li
Principal Engineer, YarCom Inc.
Please contact Dr. Chris Bataille at info@netzeroindustry.org with any question.